The ghost, finally, is really in the machine: For the first time, scientists have created neutrinos in a particle collider.
These abundant but mysterious subatomic particles are so removed from the rest of matter that they glide through it like ghosts, earning them the nickname “ghost particles”.
The researchers say that this work represents the first direct observation of collider neutrinos and will help us understand how these particles are formed, what their properties are, and their role in the evolution of the Universe.
The results, achieved with the FASERnu detector at the Large Hadron Collider, was presented at the 57th Rencontres de Moriond Electroweak Interactions and Unified Theories conference in Italy.
“We discovered neutrinos from a brand new source – particle colliders – where you have two beams of particles smashing together at very high energy,” said particle physicist Jonathan Feng of the University of California Irvine.
Neutrinos are among the most abundant subatomic particles in the Universe, second only to photons. But they have no electric charge, their mass is almost zero, and they barely interact with the other particles they encounter. Hundreds of billions of neutrinos are streaming through your body right now.
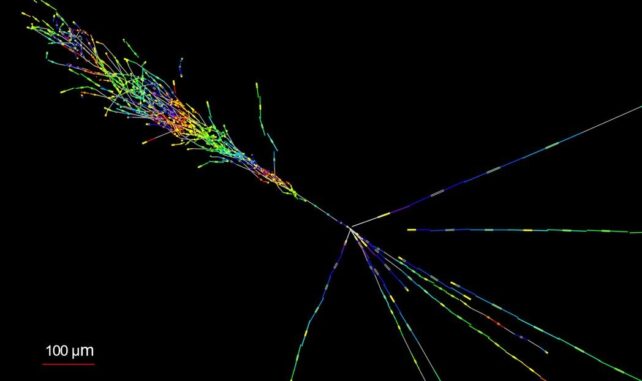
Neutrinos are produced in energetic events, such as nuclear fusion occurring inside stars, or supernova explosions. And although we cannot observe them on a day-to-day basis, physicists believe that their mass – however small – probably affects the gravity of the Universe (although neutrinos are almost excluded as dark matter).
Although their interaction with matter is small, it is not completely absent; now and again, a cosmic neutrino collides with another particle, producing a faint burst of light.
Underground detectors, isolated from other sources of radiation, can detect these explosions. Ice in Antarctica, Super-Kamiokande in Japan, and MiniBooNE at Fermilab in Illinois are three such detectors.
Neutrinos produced in particle colliders, however, have long been sought after by physicists because the high energies involved are not as well studied as low-energy neutrinos.
“They can tell us about deep space in ways we wouldn’t learn about otherwise,” says particle physicist Jamie Boyd of CERN. “These very high-energy neutrinos at the LHC are essential for understanding really exciting observations in particle astrophysics.”
FASERnu is a emulsion detector consisting of millimeter-thick tungsten plates alternated with layers of emulsion film. Tungsten was chosen because of its high density, which increases the probability of neutrino interaction; the detector consists of 730 emulsion films and a total tungsten mass of approximately 1 ton.
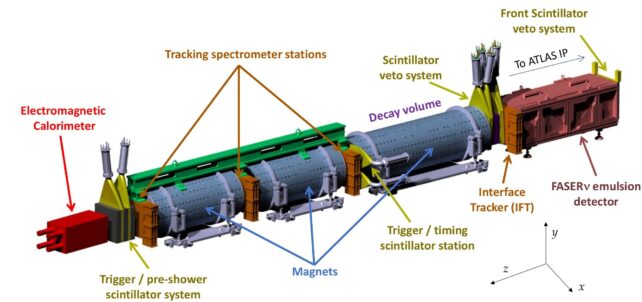
During particle experiments at the LHC, neutrinos can collide with nuclei in tungsten plates, producing particles that leave tracks in emulsion layers, just as ionizing radiation does tracks in a cloud chamber.
These plates need to be developed, like photographic film, before physicists can examine the particle trails to find out what made them.
Six candidate neutrinos were identified and published in 2021. Now, researchers have confirmed their discovery, using data from the third run of the upgraded LHC that began last year, with a significance level of 16 sigma.
That means that the probability that the signals are produced by random chance is so low as to be almost nonexistent; a significance level of 5 sigma is sufficient to qualify as a discovery in particle physics.
The FASER team is still hard at work studying the data collected by the detector, and it seems likely that many more neutrino detections will occur. Run 3 of the LHC is expected to continue until 2026and data collection and analysis is ongoing.
By 2021, physicist David Casper of UC Irvine projects that the run will produce about 10,000 neutrino interactions, which means we’ve barely exhausted what FASERnu has to offer.
“Neutrinos are the only known particles that larger experiments at the Large Hadron Collider cannot detect directly,” he says“so FASER’s successful observation means that the collider’s full physics potential has finally been exploited.”
The team results are presented at the 57th Rencontres de Moriond Electroweak Interactions and Unified Theories conference.