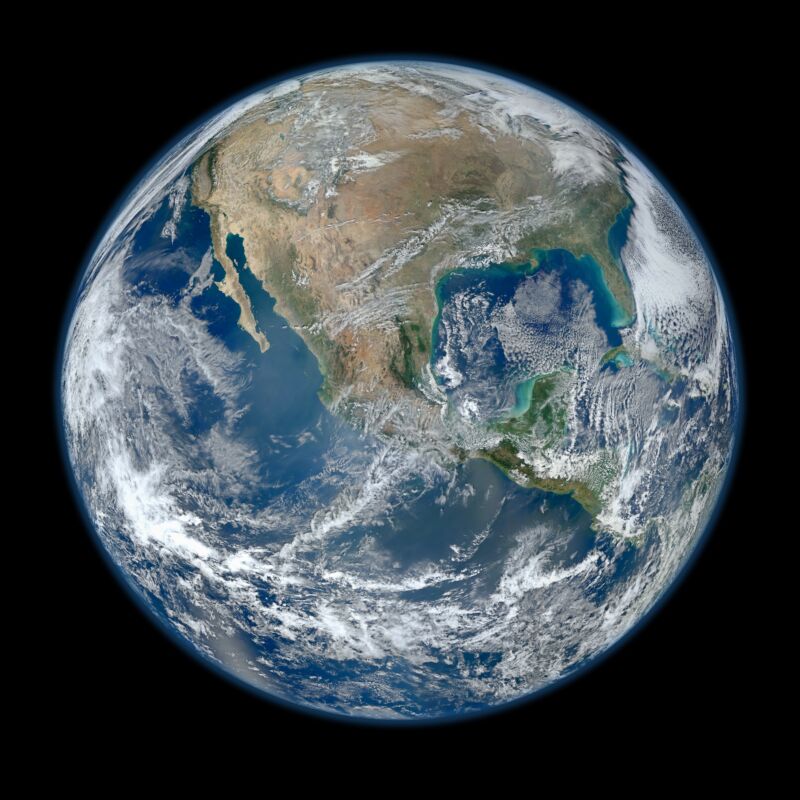
Water made Earth the planet it is—a planet known for its blue oceans. Water shapes soil through erosion and is essential to Earth’s ability to support life. But we have a hard time understanding exactly how all this water got to Earth, because the building blocks that created it were probably dry, and the collisions that turned these building blocks into a planet must have propelled any surface water into space.
Various methods have been proposed to deliver water to Earth after it was formed. But a new study takes the information we’ve gleaned from examining exoplanets and applies it to Earth. The results suggest that chemical reactions that occurred during Earth’s formation could have produced enough water to fill the world’s oceans. And, as a side benefit, the model explains the somewhat unusual density of Earth’s core.
Unreadable
Earth appears to be made primarily from materials in the inner Solar System. Not only were those materials in the right place, but the current material found in the region’s asteroids provided good matches in terms of their elemental and isotopic composition. But these materials are too dry. That’s not a surprise; the temperatures in this area keep water from condensing as a solid, as it can further into the Solar System, beyond a point known as the water “ice line”.
Any water present may have been lost to space, as the process of forming planets is thought to have occurred through collisions between smaller bodies, with the larger bodies gradually growing as the smaller ones will continue to fall upon them. Most of the water in these bodies is vaporized and potentially lost to space.
But three researchers (Edward Young, Anat Shahar, and Hilke Schlichting) focused on an additional factor that would have been present during the formation of the Solar System: hydrogen. Hydrogen is thought to have been present in large quantities early in the planet’s formation period, but was then driven away by the radiation released once the central star ignited. In our Solar System, some of these were captured by the outer planets before they disappeared. But our inner planets seem to have formed with less of the element or lost it early in their history.
But a look at exoplanets suggests that this is not an inevitable fate. We have found many rocky super-Earths that also seem to lack a hydrogen-rich atmosphere. But there is a gap at about twice the radius of Earth where we see many mini-Neptunes, which seem to maintain thick and likely hydrogen-rich atmospheres. This led to the proposal that all rocky planets start with a hydrogen-rich atmosphere and develop their first atmosphere from there. Under a certain measure, however, that hydrogen will disappear later in their history. Any atmospheres found on these planets are likely due to a secondary formation.
Taking that to its logical conclusion, Earth might as well have started with a hydrogen-rich atmosphere. So, the researchers involved in the new study decided to see what the consequences of that situation are.
Chemistry on a planetary scale
To explore that idea, the researchers essentially modeled a giant chemical reactor filled with most of the early Earth’s components and scaled up to the size of a large Earth precursor (half the size of the current Earth). These include things like iron and sodium oxides, various silicates, carbon dioxide, methane, oxygen, and more. All of this was placed under a hydrogen-rich atmosphere and heated to reveal oceans of magma created by the frequent collisions that occurred as the planets formed.
This period probably lasted tens of millions of years, in part because hydrogen atmospheres tend to retain heat very well (it can act as a greenhouse gas). This gives the chemical reactions taking place—and the researchers monitored 18 of them—time to reach an equilibrium and allows enough time for the various materials in the planetary interior to partition based on density. .
One of the things that happens is that some elements are incorporated into the iron core, including oxygen, silicon, and hydrogen. Since all these elements are less dense than iron, this has the effect of making the core less dense than if it were pure iron—something that is true of the actual Earth.
In some reactions, the addition of hydrogen involves the elimination of oxygen, and a byproduct of these reactions is water. Under the conditions explored here, the reactions produce roughly the same amount as is present in the oceans of present-day Earth. “Even though the rocks in the inner Solar System are completely dry,” the researchers wrote, “the reactions between H2 Atmospheres and magma oceans will produce large amounts of H2O. Other sources of H2O is possible, but not necessary.”
The limitations of modeling
On the plus side, the simulations work over a wide range of temperatures—all it needs is enough heat to keep the planet melting while the processes described here reach an equilibrium. It also works for different precursor sizes, but fails if the precursor is too small. That corresponds to the extreme dryness of Mars and Mercury. The main variable ends up being how much water is produced; if more hydrogen went into the core, it could easily create a water world with three times the volume of today’s oceans.
Although the model is robust to many changes in initial conditions, it is limited by an incomplete picture of the chemistry of the early Earth. Sulfur and nitrogen, which played key roles in Earth’s chemistry, are noticeably missing.
But the big gap in the model is what happens after the water forms. Because of the presence of the magma ocean, it will end up in the atmosphere, where it can be broken down by solar radiation and disappear if the Solar System’s hydrogen is depleted. The same is true for any future impacts that heat the planet, such as the giant collision that formed the Moon. If there is enough hydrogen around, this is not a problem because water can simply change. And the researchers cite research showing that a water-rich atmosphere could survive even a massive collision. Finally, you can imagine the conditions under which there was an initial overproduction of water, but enough was lost through these processes to leave the Earth in its current state.
So, while water production does not require any fine-tuning of conditions, its maintenance may.
But the implications for worlds beyond ours seem somewhat greater. These results suggest that a large range of initial conditions must have produced water during the formation of rocky planets. So, if we consider planets in exosolar systems, it may be a question of wondering if they experienced conditions that would cause them to lose water rather than if they had it in the first place.
Nature, 2023. DOI: 10.1038/s41586-023-05823-0 (About DOIs).